The original language release (Italian) is the official and authorized version of the release. This translation is only a means of assistance and should be compared with the original language text, which is the only version of the text that will be legally valid.
This article was published by ASCCA News – the house organ of ASCCA – Association for the Study and Control of Environmental Contamination.
Highly Potent Active Pharmaceutical Ingredients (HPAPIs) are a rapidly expanding segment of the global pharmaceutical industry. Currently, over 1,000 highly potent small molecules are under development, with market estimates predicting an annual growth of about 10% in this sector. By the end of 2022, over 40% of all drugs in development fell into the high activity category. Recently, HPAPIs in the form of Antibody-Drug Conjugates (ADCs) have further boosted market development. Managing HPAPIs in pharmaceutical manufacturing requires appropriate solutions to ensure worker safety and health, support contamination control, and production sustainability. This article illustrates the integrated approach of the Containment Mix (CM), outlining its contents and illustrating some advantages through application examples that link results and considerations to the elements set out in the paradigm itself.
Introduction
HPAPIs can be defined based on pharmacological activity parameters, such as a daily therapeutic dose of ≤10 mg, an occupational exposure limit (OEL) of ≤10 µg/m³ TWA, high selectivity, and/or potential to cause cancer, mutations, developmental defects, or reproductive toxicity. Alternatively, they can be defined by the innovative nature of a compound whose potency and toxicity are not yet fully known. In the late 1980s, a group of pharmaceutical companies began defining hazard categories for active pharmaceutical ingredients. Over the following decades, this system was further developed, delineating these categories more comprehensively, now commonly known as Occupational Exposure Bands (OEB). There is no internationally harmonized standard for this categorization: it usually comprises 4 to 6 categories, each an order of magnitude, where less potent substances are assigned to the first category, and more potent ones to the highest category. An example of these categories is shown in Figure 1. If an OEL cannot be established for a substance with the available data, it can be placed in one of the potency categories and managed according to the controls it requires. This classification can be adapted based on the substances to be produced, the strategy, and the measurement results, such as those obtained with a Strategic Containment Control Program (PSCC), which will be discussed later.
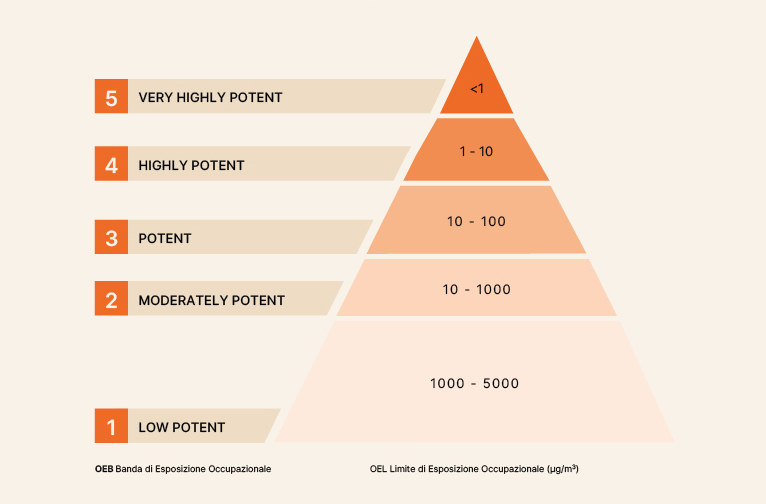
Figure 1 – Example of Occupational Exposure Categories Classification
We will also see how the approach to containing substances or chemical agents (particularly HPAPIs produced in bulk or processed into specific formulations, the subject of this article) is presented as the contribution of a series of elements (the Containment Mix), of which the PSCC is part and at the same time the driving force for countering, controlling, and limiting the spread of these substances in the production environment and, more broadly, in the internal and external environment of the facility.
Methodology
When discussing the containment of highly potent active ingredients in all phases of industrial and laboratory production, the question should not be whether containment is necessary, but rather what degree of containment is required for each context. This approach shifts the focus from the use of technologies per se, depending on the contingent context, which is difficult to justify to users and regulatory authorities, to the adoption of integrated strategies consistent with the strategic approach and corporate culture. The growing adoption of control banding approaches within the chemical and pharmaceutical industry, with the definition of specific pathways for managing substances based on their hazard level, further suggests the importance of investigating containment strategies for all active ingredients and in all processes, whether related to production, research, quality control, logistics, or maintenance. This article introduces the Containment Mix (CM) as a paradigm of a comprehensive approach, outlining its contents and illustrating some advantages through application examples that link results and considerations to the elements set out in the paradigm itself. The CM emphasizes the interdisciplinarity of the company’s skills and functions, demonstrating how they can contribute to achieving a higher goal compared to an approach that does not integrate the various aspects so broadly. A schematic representation of this approach is shown in Figure 2.
Elements of the Containment Mix
• Process Evaluation and Development
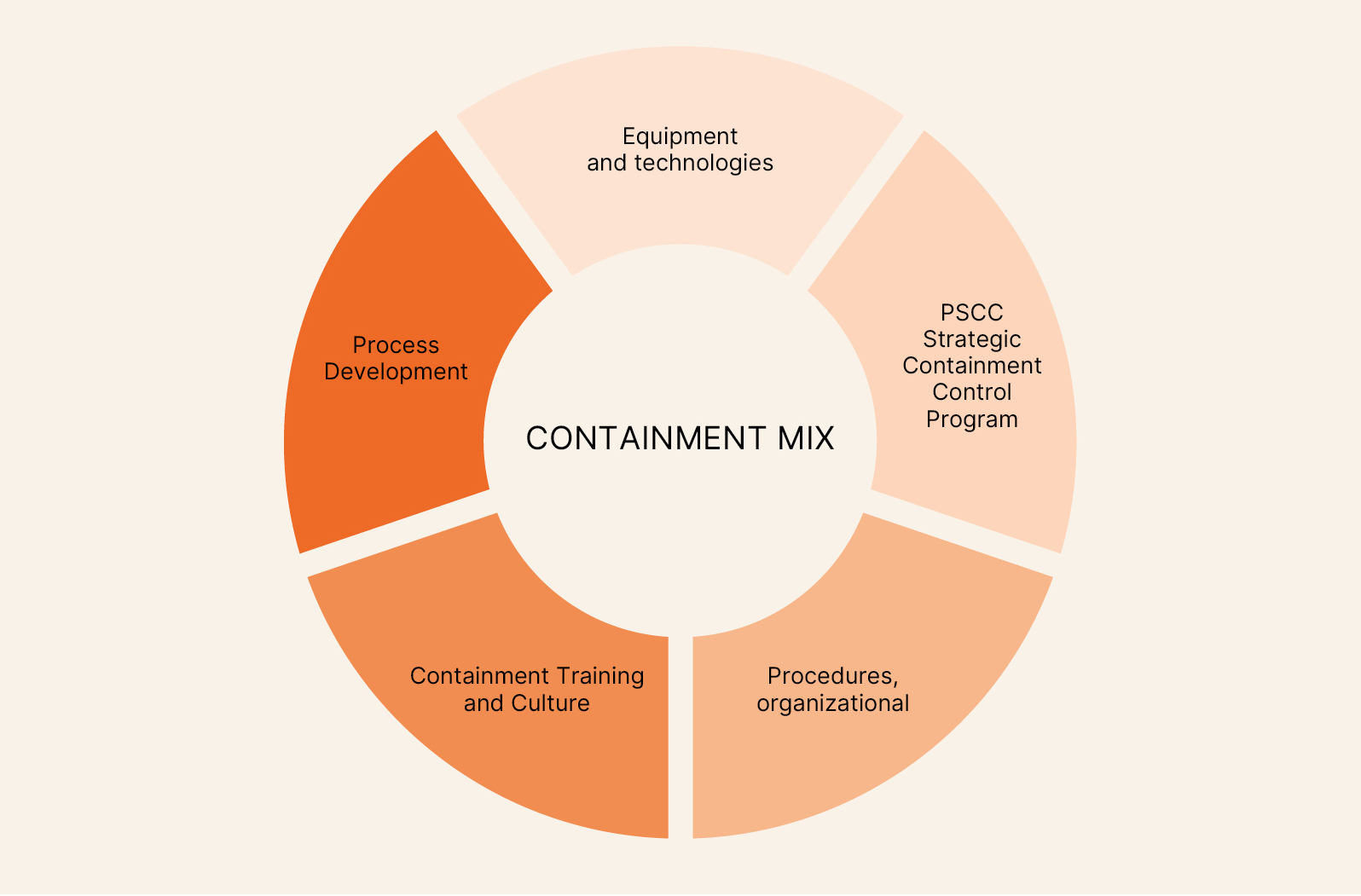
Figure 2 – The Elements of the Containment Mix
• Containment Training and Culture
• Strategic Containment Control Program (PSCC)
• Procedures, Organizational Controls, and PPE
• Equipment and Technologies
Each of these elements, as demonstrated by a wide range of measurements in real cases, has a significant and decisive contribution to exposure control. Applying the elements of the CM requires guidance that continuously monitors the evolution of banding, substance properties, equipment, procedures, and training throughout the process lifecycle. As illustrated later, this can be implemented through the PSCC.
Process Evaluation and Development
During process development, essential activities are carried out to identify, mitigate, and possibly eliminate risks associated with the substances involved, selecting appropriate reagents and their combinations, acquiring data on chemical risk and limits (OEL), and deepening process design to manage these risks effectively. There are many risk-based approaches to refining processes during the development phase to reduce exposure risk, and it is crucial that their feasibility is analyzed from the early stages. The result of this assessment could lead, for example, to eliminating or replacing highly toxic solvents, using reagents or intermediates in suspension in certain loading or transport phases, optimizing process flow to ensure that transfers between containment equipment are minimized. In specific cases, technicians may be able to reduce the number of synthesis steps, thus eliminating isolated intermediates and the associated exposure risk.
Equipment and Technologies
The use of engineering controls and equipment to achieve containment objectives is well documented in the literature. These are also known as collective protection measures as they protect everyone in the work area, unlike personal protective equipment (PPE) that individually protects the wearer. Primary containment systems refer to those engineering controls that limit the spread of a substance from the contamination source, usually within the equipment itself. Secondary containment refers to measures that limit the spread of the substance in the production area that may have escaped primary containment. Examples of primary containment measures include isolators used for handling HPAPIs, continuous liners, and other interfaces, oral solid dose (OSD) production machines, and transfer systems. Examples of secondary containment measures include cleanrooms, airlocks, and pressure gradients between the outside and inside of the production area. The most protective approach in terms of operator safety, but also with interesting implications for contamination and sustainability, remains containment “at the source,” favoring primary systems and appropriately regulating the choice of secondary ones based on these. There are two common aspects, emerged from years of professional practice, to avoid when discussing equipment: the first concerns the tendency to base the entire containment strategy solely on their introduction, neglecting the importance of other CM elements. Secondly, it is often seen that the need to test equipment with a procedure representative of the actual operations to be conducted within them is not recognized, relying on inaccurate tests and “generic” procedures that generate incorrect, often misleading data.
Containment Training and Culture
A “risk-aware” containment culture is crucial for the program’s success. It is essential that organization members at all levels clearly understand roles and responsibilities to ensure safe containment of HPAPIs. Management should demonstrate strong commitment and lead the program’s implementation, ensuring that necessary resources are allocated for containment systems, from design to scheduled maintenance and continuous PSCC maintenance. Operators should feel responsible not only for themselves but also for their colleagues, ensuring the correct use of containment systems; they should also be encouraged to continuously identify and implement improvements to these systems, discussing them directly with management. Data collected over years of assessment and testing have shown that personnel expertise and experience can significantly increase equipment containment levels; therefore, an effective safety training program for HPAPI production should be adopted and made mandatory with a certain frequency for all operators, staff managing the Containment Control Program, and other individuals interacting with these substances. The continuous training plan should always include general topics on containment principles and occupational hygiene, mainly aiming to understand the nature of risks associated with using potent compounds in all operational phases, from routine operations to maintenance, cleaning, failures, and emergencies. Since visual inspection is not sufficient to detect unacceptable exposure situations (safety levels are usually well below the visible threshold), effective training should also delve into the characteristics of these substances, explaining what happens at these hazard levels in terms of possible emission and diffusion pathways, thus highlighting the importance of correct practices and even complex containment measures. Finally, regarding equipment, adequate training should include not only a precise verification of machine, tool, and interface usage methods but also an in-depth analysis of the critical points of the main containment technologies present. Recently, virtual tools in augmented reality have been developed, making it very effective to use targeted training for a device or simulate a specific operational or emergency management task.
Strategic Containment Control Program
(PSCC) As already introduced, the CM includes a fundamental element, the Strategic Containment Control Program (PSCC). It is a sort of direction for strategies, evaluations, measures, results, and control actions necessary to ensure that the containment objective is constantly achieved and maintained over time. It is advisable to formalize the PSCC in a structured program, with defined guidelines, conducted by a working group with diverse skills, such as occupational hygiene, toxicology, risk assessment, analytical chemistry, quality, and environment. This team could include both internal company figures and external experts. The PSCC adopts a risk-based approach to containment that addresses the entire lifecycle of processes, as shown in Figure 3.
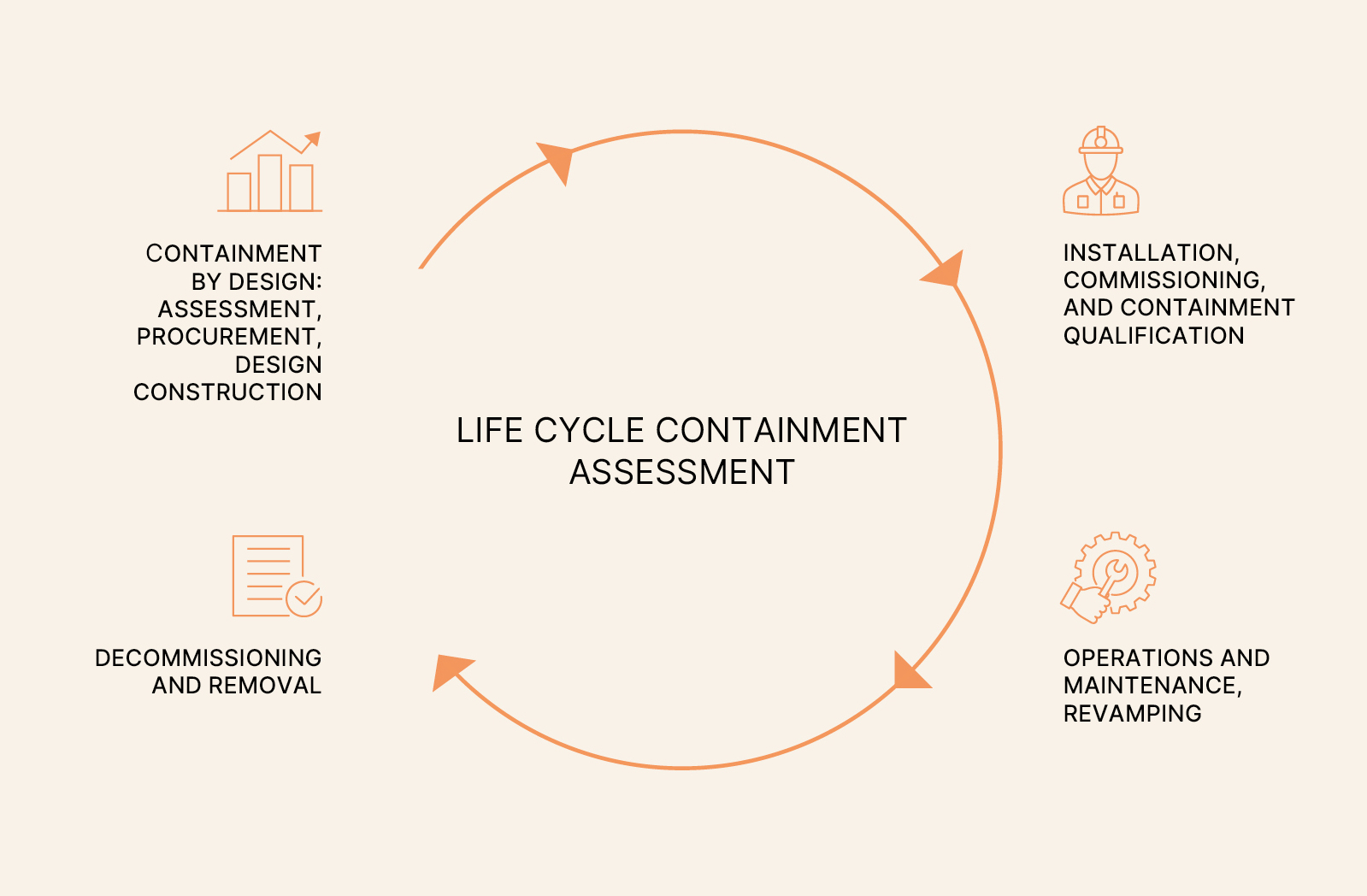
Figure 3 – The Containment Life Cycle
The focus of the program should be as broad as possible and include substances, processes, equipment and protective devices, OEL, OEB, and exposure risks, procedures, and monitoring tools, evaluated according to robust industry references and in line with the control banding strategy in use. The activity should progressively cover the new design or periodic reassessment of all production, research, quality control, logistics, and maintenance processes, considering all routine and non-routine activities, both productive and non-productive.
The containment evaluation cycle for new implementations or revamping, for example, conducted in this manner, provides a fundamental basis for design from the outset, also facilitating technical procurement and defining requirements for technology suppliers. In the subsequent commissioning and qualification phase, it will be essential to obtain proof of actual performance through measured data and samples collected during standardized and reproducible monitoring (as described in the ISPE SMEPAC methods).
Given the importance of comparing results over time and comparing different technologies, departments, and distant facilities, the concept of reproducibility here assumes even greater value, making the use of standardized test methods conducted within a program capable of providing adequate evaluation indispensable. The operational phase of the processes that follows can vary in duration, from days to years, depending on the type of devices used (for example, flexible glove boxes are usually employed for short periods) and the productive life of the active ingredient, which varies significantly between research and development, clinical phase formulation, and large-scale production. Even in this phase, the containment test (SMEPAC), performed with a certain frequency based on available resources, the adopted strategy, and previous results, is essential to confirm through real measurements the maintenance of control over equipment, procedures, and protective devices. Combining the frequency of these tests with a robust industrial hygiene monitoring plan, focused on exposure to active ingredients in activities considered relevant in the risk assessment, ultimately achieves the maximum possible control over contamination and the consequent potential exposure. In the final phase of equipment decommissioning, attention focuses on the operational methods for dismantling or disassembling the systems, which must be evaluated and, if necessary, improved procedurally or technologically. A case of particular interest due to its high criticality and frequency in this phase is the decommissioning of single-use containment systems (data collected shows these tasks are usually at higher exposure risk than the actual operation).
Below are some tools useful for the development and maintenance of the PSCC:
• Determination of PDE (permissible daily exposure) and OEL;
• Determination of acceptable surface contamination limits (ASL);
• Development of analytical and sampling methods for determining HPAPI in the air, on surfaces, and in other matrices;
• Qualitative/semiquantitative risk assessments in various formats focusing on exposure risk, equipment criticality, processes, environment, etc.;
• Quantitative containment tests with surrogate (SMEPAC);
• Occupational hygiene monitoring campaigns;
• Methods for inactivation and/or decontamination of APIs.
Application Examples
Here are some examples from scientific consulting and testing activities carried out in various HPAPI research and production settings to understand why it is useful to apply this approach.
Research and Development and Quality Control Activities
A first example is the assessment and testing of laboratory activities conducted in recent years in some Research and Development and quality control departments of existing facilities. Here, the risk assessment highlighted the main determinants of exposure and contamination by identifying the types of equipment, the quantities and physical state of active substances, the severity of operations, and the operating methods. Some typical criticalities of these activities brought to light include the need to evaluate new chemical entities (NCE), multistep processes that are not always standardized, the use of equipment not always designed for containment, and less segregation of areas compared to production. In this scenario, some CM components such as training, operating methods, and containment culture played a key role. The results led to a statistically adequate characterization of equipment and unit operations according to the control bands used for laboratory activities, allowing for the management of substances mostly in the OEB3 and OEB4 ranges. The data also allowed for identifying even better performance in cases where the physical state of the active substance (e.g., liquid) or the severity of the operation represented a “best case,” or, conversely, to direct choices towards partial or total segregation of activities (solid state substances or with greater severity and potency). In cases where laboratories had a separate unit for handling highly active substances, it was possible to precisely define the applicability of these areas in terms of OEB/OEL and maintain containment control, revising operating methods over time according to the evolution of activities. From a lifecycle containment perspective, the data highlighted some differences between modern and obsolete systems and allowed for planning multi-year monitoring organized based on the priority of the most critical situations.
Production Activities with Flexible Isolators
The second example concerns the use of flexible isolators in production for containment operations such as dispensing, formulation of clinical batches, and preparation of HPAPI for sterile filling. These systems, made of transparent and conductive polymer material, are mostly used for substances up to the OEB4 category, depending on whether they are passive or maintained internally at negative pressure. Single-use technologies offer advantages in terms of cost and application flexibility, easily adapting to existing plants and structures to improve processes in a targeted manner. In multiproduct contexts, where rapid turnover between products is required, the single-use approach reduces or ideally eliminates the need for cleaning verification. In various experiences conducted on flexible systems, the evaluation was applied from the early stages of the project (containment design) to offer adequate containment performance in all intended uses, continuing with an in-depth consideration of the equipment lifecycle. The lifecycle of this type of containment system is much shorter than their fixed counterparts, and cleaning and decommissioning operations become almost routine in terms of frequency. Measurement results showed that many systems depended on the operator’s skills and behaviors and that some phases of use, especially non-productive ones such as cleaning, collapsing, and detaching from the structures to which they were integrated, showed significant criticalities in terms of containment. The program allowed in many cases the alignment of CM elements with the specific characteristics of this technology. This improved and maintained control over various peculiar aspects of operating with such systems, such as the need for frequent training, complex and sometimes not fully defined operations, significantly shorter lifecycles, and decommissioning operations performed almost routinely.
Production Activities in High-Containment Isolators
The last example describes an HPAPI synthesis activity on a kilogram scale in dedicated suites served by systems of very high containment complexity and performance (OEB5, range 0.01-0.001 μg/m³). Secondary containment consists of a segregated area with separate unidirectional accesses for materials and personnel, while the process and primary containment were developed in parallel, creating an “all-in-one” system that integrates all reaction steps into a single piece of equipment. Activities are carried out entirely under high containment in a series of interconnected work chambers served by passboxes with multiple Continuous Liner System (CLS) interfaces. This configuration eliminates the problem of transferring between different process isolators and the consequent potential contamination that could result. At the same time, it allows the system to be used modularly, using independent parts and in/out interfaces. CIP/WIP was integrated into the equipment design, minimizing the need to extract parts outside and allowing for cleaning restoration of the machine and internal equipment almost entirely in containment. The first phase involved an in-depth analysis of all unit operations of the synthesis steps that can be performed with the system, as well as a verification of the department’s operating methods, including internal and external activities to the isolated system up to the corridor limits. The analysis included both routine and non-routine, productive and non-productive operations, breaking them down to the tasks relevant to containment. Subsequently, it was identified which activities were a priority for quantitative containment and potential exposure evaluation, to be carried out using the tools illustrated in the methodology. Quantitative evaluation has been continuously conducted for years following a multi-year plan and using a combination of surrogate containment tests and industrial hygiene monitoring. Containment tests focus mainly on potential emissions from primary and secondary containment systems, while monitoring focuses on possible operator exposures. Both approaches required a significant preparatory phase, aimed on the one hand at adapting sampling and analysis methods to the containment degree to be tested and on the other at validating active determination methods capable of reaching the required verification limits. Over the years, the program has maintained continuous containment control based on acquired measurements, bringing optimizations in operating methods, interface use, analysis methods, investigation points, and additional protective devices compared to primary containment.
Conclusions
The production and formulation of HPAPIs is a continuously developing sector that poses significant challenges for worker safety and health, necessitating an advanced approach to containment. It is essential to understand that an effective containment program does not limit itself to engineering controls but integrates these with cultural, organizational, and procedural elements of the Containment Mix (CM) throughout the entire lifecycle. This ensures efficiency, flexibility, and adaptability in production processes, maximizing the success of investments and limiting over-engineered solutions. This approach is particularly useful for reconsidering conventional productions, such as API synthesis or oral solid dosage forms originally designed when the hazard index of substances was an order of magnitude lower. The strategic Containment Control Program is based on a risk-based approach and field verification through comparable, repeatable measures collected where necessary and with the highest possible accuracy. Data from SMEPAC containment tests and industrial hygiene measurements, collected within a reliable and monitored program over time, are fundamental for employee safety and can contribute to the assessment of cross-contamination risk with other products. In terms of sustainability, an integrated assessment can contribute to better balancing primary containment technologies and secondary ventilation, optimizing the need for PPE and cleaning processes. As seen in the application examples, this can lead to resource savings, including energy, materials, and water. Reducing PPE use and optimizing cleaning processes, guided by structured and informed data, also helps reduce environmental impact, both in terms of waste generation for incineration and the amount of active ingredient released in the plant’s liquid and gaseous effluents. Ultimately, the development of HPAPIs is based on minimizing the amount of drug needed to achieve the same therapeutic result, developing substances that inherently require a strategic Containment Control Program. This type of control should generally always be sought and applied not only to high-containment systems or extremely potent active ingredients. Its positive impact can be broad, covering process aspects, operator protection, support for a contamination control strategy, and finally, countering the spread of chemical substances in environmental matrices.
Highly Potent Active Pharmaceutical Ingredients (HPAPIs) are a rapidly expanding segment of the global pharmaceutical industry. Currently, over 1,000 small molecules highly powerful are under development, and market estimates predict annual growth of approximately 10% in this sector. By the end of 2022, more than 40% of all drugs in development were re-entry in the high activity category. A further impetus to the development of the market has been given in more recent times by HPAPI in the form of the so-called ADCs (Antibody-Drug Conjugates). The management of HPAPIs in pharmaceutical manufacturing requires appropriate solutions to ensure the safety and health of workers, as well as to support contamination control and production sustainability. This article illustrates the integrated approach of the containment mix (CM), outlining its contents briefly and illustrating some advantages through application examples which, as can be seen, link results and considerations to the elements set out in the paradigm itself.
This article was published by ASCCA News – the house organ of ASCCA – Association for the Study and Control of Environmental Contamination.
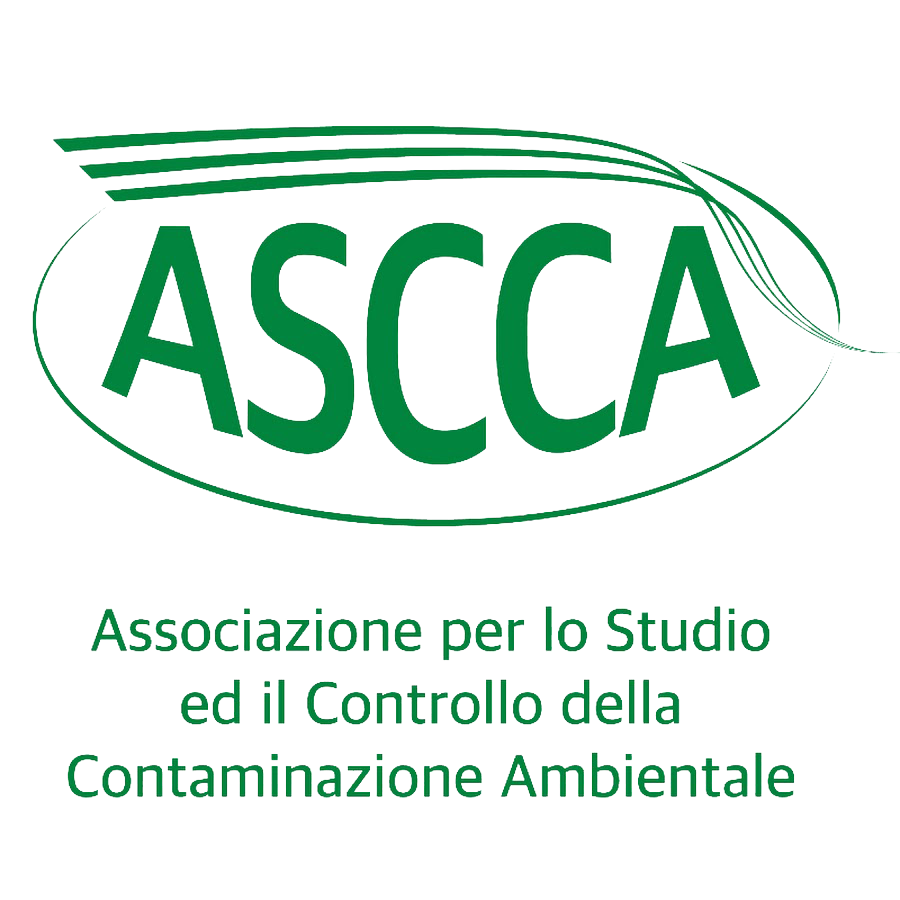
Articoli correlati
March 25, 2025
Sterilization Processes
The goal of any sterilization process is to destroy microorganisms present on a…
February 27, 2025
The evolution of the SMEPAC guide
This article was published by ASCCA News. A new edition of the guideline for…